Let’s pause to appreciate nuclear medicine
Neutron factories are keeping us healthy, but the field needs more attention
Nuclear medicine is one of those cool specialities that doesn’t get enough attention. While nuclear energy has enjoyed a revival in recent years, little attention has been paid to nuclear medicine. That’s probably partly because explaining it in technical terms inevitably removes some of the magic and partly because many people fear radiation and don’t want to think about it.
But nuclear medicine deserves attention. Not all of the world's nuclear reactors are used for producing energy; they are also used for producing radioisotopes for medicine and industry, training, and other purposes. They are known as research reactors, and there are currently around 220 research reactors in 53 countries. In the heyday of nuclear development, in 1975, there were 373 neutron factories in 55 countries.
Developed in the 1950s by physicians who initially used it to diagnose and treat thyroid disease, nuclear medicine can now help treat myriad health issues by using nuclear imaging to assess bodily functions, diagnose problems and treat diseases. It is a core ally in the fight against poor health.
X-rays are generally accepted as cool technology - there are countless superheroes with X-ray vision as a superpower - but I don’t know of any superheroes who can do what nuclear medicine is capable of. Nuclear medicine scans are in a league of their own.
How do they work?
Nuclear medicine uses radioisotopes, which are produced by nuclear reactors. Radioisotopes are naturally decaying atoms with unique qualities that make them useful for treating different health issues. Radionuclides, also known as radioisotopes or tracers, are very small amounts of radioactive substances produced by reactors that are used in diagnostic imaging.
Here comes the cool bit. From a chemical perspective, every organ in our bodies acts differently, and specific organs absorb specific chemicals. For example, the thyroid absorbs iodine, and the brain consumes glucose. Radiologists can attach different radioisotopes to biologically active substances to obtain information about organs. The tracers are absorbed by body tissue, and the minuscule amount of radiopharmaceutical reveals a mass of information before it decays. After the radionuclide has collected in the body tissue being examined, radiation is given off. A detector then picks up this radiation using a gamma camera. Tracers are tracked using specially designed cameras, the two most common of which are Single Photon Emission Computed Tomography (SPECT) and Positron Emission Tomography (PET) scanners.
Why is this important? Soft tissue like intestines, muscles, and blood vessels are hard to see on standard X-rays. While other imaging techniques like CT scans and MRIs can gather anatomical data, nuclear imaging shows us how organs and tissues function from outside the body, including organ and tissue structure and function, and how well the organ or tissue is working.
It’s also a non-invasive, pain-free procedure, which (drumroll please) leaves no trace.
Life-saving technology: a crossroads
Every year, at least 30 million people are helped by nuclear medicine, including 10 million patients in Europe. According to the IAEA, in industrialised countries, three out of every four patients benefit from some form of nuclear medicine.
However, access to nuclear imaging is not available everywhere in the world, and although low-income countries suffer the worst, there are also some industrialised nations that fail to take full advantage of this technology.
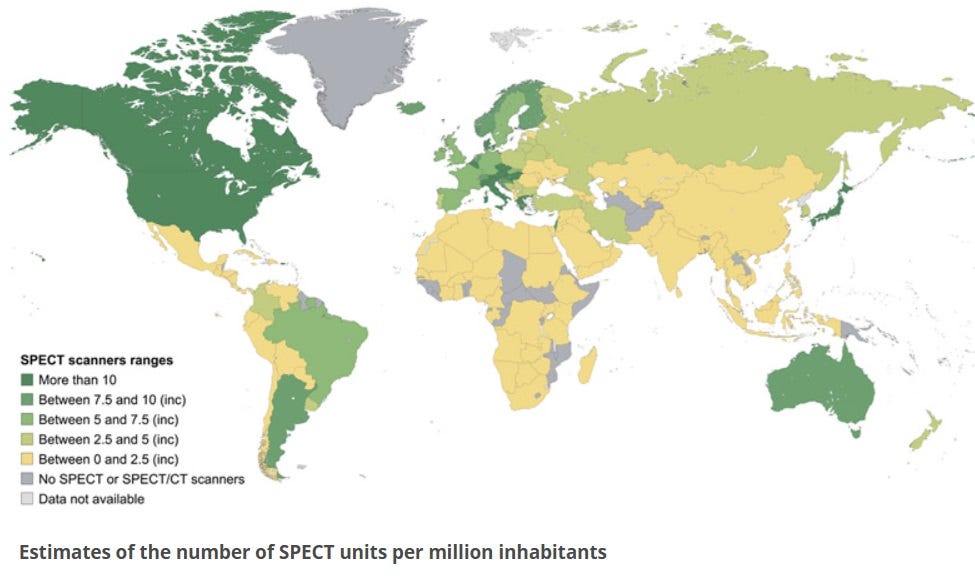
It’s hard to say why, but certainly the historically negative public perception of nuclear power is an obvious factor since boosting nuclear medicine at home means building reactors. The cognitive dissonance that surfaces at the mere mention of nuclear technology has led to strange policy decisions globally, like Australia’s ongoing ban on nuclear energy while also being one of the few nations in the world that produces the radioisotopes necessary for nuclear medicine procedures through their research reactor ANSTO.
In the UK, this dissonance has resulted in stagnation in the field of nuclear medicine, as the country could run out of life-saving nuclear substances needed for this life-saving technology. Britain was once a leader in the world in the field of nuclear medicine, but it has fallen far behind. The UK's current supply chain for nuclear medicine is vulnerable, as we have no home-grown supply for the vast majority of radioisotopes needed, and rely on imports from ageing European facilities instead.
A core problem with relying on exports, besides the obvious points of vulnerability and lack of sovereignty, is that radioisotopes are highly perishable. Once produced, they have to be processed, shipped and used within a short period, sometimes on the same day or within a few days. Any delays or disruption to the supply chain can prove costly and deadly.
The good news is that the Welsh government wants to address this supply chain dependency and has proposed building a new laboratory to supply radioisotopes for the UK with a medical isotope reactor called the Advanced Radioisotope Technology for Health Utility Reactor (ARTHUR). ARTHUR is currently undergoing a feasibility study to obtain development consent. The clock is ticking, and the pace is slow.
Sadly, UK governments have known for some time that nuclear medicine is on a knife edge - twenty years ago, experts issued a warning that nuclear medicine services were ‘close to collapse’ in the UK. When I spoke to Professor Simon Middleburgh, who researches nuclear materials at the Nuclear Futures Institute, he described the situation in Britain as “about to fall off a cliff edge. Our current reactors are too old, and the UK is already bad at using nuclear medicine to diagnose people. If we don’t invest in nuclear medicine now, it will result in more deaths than from COVID.”
Without ARTHUR, he warns, in the UK, “nuclear medicine will turn into a thing for only rich people”. He is also concerned that anti-nuclear activists will try to stop or delay the new facility being built. This story is not dissimilar to that of nuclear energy in the UK, and similarly, one of the criticisms of nuclear medicine is that it generates radioactive waste. Although this is low-level waste, mostly in the form of used personal protective equipment, it still requires effective waste disposal, which has historically been protested by persistent anti-nuclear activists.
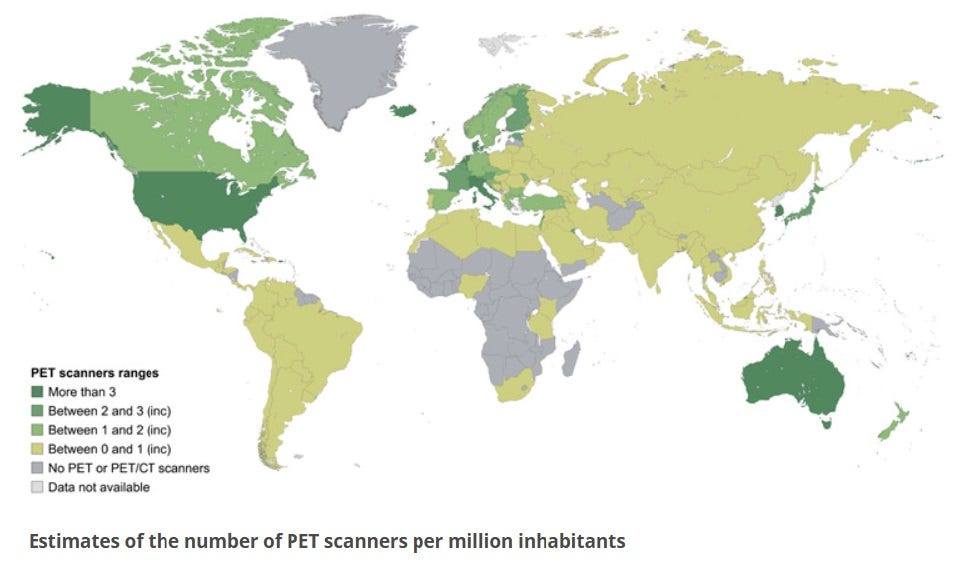
But - Luddites aside - the success of nuclear imaging development in Europe deserves recognition. Some of the best universities to study nuclear medicine in the world are in the UK, and European researchers and businesses have developed some highly innovative cancer treatments, which offer much better and safer alternatives to other existing treatments.
We are now at a crossroads with nuclear technology. Europe needs to prioritise the supply of radioisotopes to increase patients’ access to nuclear medicine. This will require investment, increased cooperation, and the removal of regulatory barriers to the supply of radioisotopes, including reducing dependency on third countries for raw materials.
Meanwhile, the US performs roughly 20 million nuclear medicine procedures annually, which is about half of the world's total. In the US, Middleburgh tells me, it’s routine to use nuclear medicine on pets. However, a paper last year drew attention to the challenges of sustaining nuclear medicine in the US due to a lack of expertise, notably physicians, both in academia and in private practice. Building reactors and ensuring import supplies is one side of the coin, and the other is ensuring that here are enough qualified specialists to operate the equipment.
Outside of the wealthier nations, there is a significant shortage of equipment and workforce for nuclear imaging around the world, and one study found that:
A comprehensive scale-up of imaging, treatment, and care quality would avert 9·55 million (12·5%) of all cancer deaths caused by the modelled cancers worldwide, saving 232·30 million life-years. Scale-up of imaging would cost US$6·84 billion in 2020–30 but yield lifetime productivity gains of $1·23 trillion worldwide, a net return of $179·19 per $1 invested.
Healthy people benefit humanity. For those living in the poorest nations to gain access to improved healthcare, nuclear medicine will play a vital role going forward, although when and how that will happen remains to be seen. It would be wise to get our own houses in order, before trying to tackle the wider problem.
Relying heavily on exports for essential life-saving equipment is always problematic, but perhaps even more so with increasing geopolitical instability. World leaders and industries need to make plans to retain the ability to produce life-saving radioactive substances. The challenges are not insurmountable, but they are multitudinous. Acting efficiently and promptly means investing in the infrastructure of radionuclide production (aka building new research reactors), taking measures to recruit and train the next generation of specialist workers, and developing long-term programs to sustain nuclear medicine research. Then we can all enjoy the benefits of better healthcare and longer lifespans for many years to come - including for our pets.
A very good commentary by Zion on the issue. I would only add that some medical isotopes come from an assortment of particle accelerators. An example of this is TRIUMF in British Columbia as an adjunct to the University of British Columbia.
https://www.triumf.ca/
The impact of nuclear medicine over the past century has been enormous. It has allowed visualization of the human body interior in ways never imagined in the 19th century or before. Today nuclear medicine avoids the need for an enormous number of procedures in exploratory surgery. It should be noted that one of the greatest scientist of all time, Marie Curie, was heavily involved in nuclear imaging. WW1 was the first time that X-Rays were used for examination of injuries. Curie was very active as a radiologist volunteer with the French army medical services providing X-Ray examinations to wounded soldiers. These first generation X-Ray machines were extremely poor in protection operators from nuclear doses. And the doses were very, very large. Wartime X-Ray exposure may account for most of Marie Curie's lifetime radiation dose and not her laboratory work in radioactive materials.
Marie Curie may be the greatest scientist who ever lived. Her two Nobel Prizes, 1903 for Physics and 1911 for Chemistry, make her the only double winner of a Nobel Prize in two different disciplines. All this and her volunteer medical work in WW1 meant a career that none can match, before or since.
There are als the NDT applications that use Co-60 made in nuclear machines to keep built steel structures safe, including ships and other heavy metal stuff. Oil and Gas platforms? Also used in Radiotherapy machines and the sterilisation facilities used in food processing and medical dressings, etc. Plutonium powered the Voyager exploration vehicle, Pu-238, not fissile but has an energetic decay. I imagine Wade Allison could give you chapter and verse.
PS Much realer science than JSO's. Much respect for transitioning to reality.